Silicon Anodes – The Future of Batteries?
For decades, society has relied on graphite-based anodes in lithium-ion rechargeable batteries. Their relatively high energy density, long cycle life, and low volume expansion under charging and discharging have made them the main player in batteries. However, as society continues to transition to sustainable energy, the need for higher-performing batteries becomes more and more important.
One of the graphite-based anode’s primary contenders is the lithium-silicon anode. The first lithium-silicon electrochemical cells were tested back in 1976 by General Motors, seven years earlier than the first graphite anodes. Sila Nanotechnologies, a material company dedicated to improving energy storage performance and production efficiency, states, “[New energy storage technologies] will be driven by the demands of electric vehicles (EVs), further accelerated by autonomous vehicles and storage for intermittent renewables.” Recently, studies have theoretically calculated that silicon anode batteries have specific capacities of over 4,000 mAh/g, compared to the graphite anode’s 400 mAh/g.
The more than tenfold increase in energy density has led many scientists to explore the feasibility of doping graphite anodes or even replacing them with silicon. As graphite-based anodes have almost reached their theoretical limit, companies like Sila are looking into implementing silicon as a way to continue pushing society forward. Currently, the costs of lithium-ion batteries average around $100/kWh, but Sila predicts that, with novel chemistry and manufacturing with silicon, that price could drop to $50/kWh. Currently, scientists have been using different techniques, such as cyclic voltammetry or galvanostatic charge-discharge to simulate specific capacity and cycle life in real-world environments to gauge silicon’s likelihood of coming to mass production.
However, there are some drawbacks to lithium-silicon batteries. In the charging and discharging phases, silicon has a tendency to expand and shrink to a great extent: almost 300% in charging according to Pacific Northwest National Laboratory, compared to graphite’s 6-20% average expansion. When the anode changes size, it can lead to cracking in the solid electrolyte interface (SEI), which is crucial to the performance of a battery. Additionally, pure silicon lacks the ability to function at such a high level for prolonged periods of time. To combat these limitations, scientists have been testing doping graphite with small amounts of silicon to reap the benefits of both elements.
All in all, silicon is generating considerable attention as battery manufacturers hope to change the energy industry for the better and make silicon the dominant chemical element in battery manufacturing.
Your donation will support the student journalists of Dublin High School. Your contribution will allow us to purchase equipment and cover our annual website hosting costs.
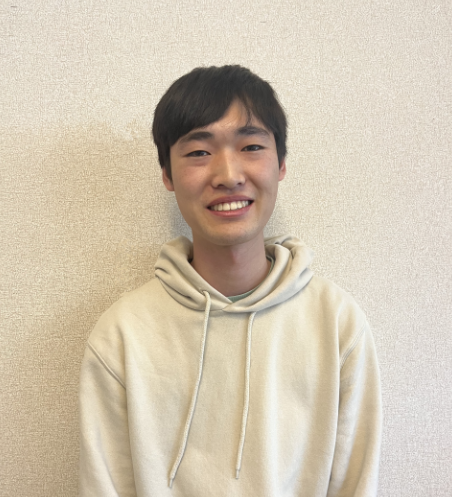
Alexander Suen is a Senior at Dublin High School. He enjoys writing about various topics that are popular and relevant. In his free time, he likes...